
Prev Page |
Home Page
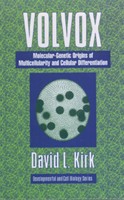
David L. Kirk
VOLVOX, Molecular-Genetic Origins of Multicellularity and Cellular Differentiation
1998
Cambridge University Press
ISBN 0-521-45207-4
CONTENTS
Preface
Prologue 1
1. Introduction 8
1.1 Multicellularity and cellular differentiation have evolved many times 8
1.2 The evolutionary origins of most forms of multicellularity are obscure 12
1.3 Desirable features of a model system for studying the origins of multicellularity 15
2. The volvocales: many multicellular innovations 19
2.1 The green algae: an ancient and inventive group 20
2.2 Chlamydomonas and its relatives: master colony formers 23
2.3 The volvocine algae: a linear progression in organismic complexity? 26
2.4 The volvocacean family tree appears to have a complicated branching pattern 28
2.4.1 A single volvocacean species name can mask extensive genetic diversity. 28
2.4.2 There are marked differences in developmental patterns within the genus Volvox 30
2.4.3 Molecular evidence indicates that most volvocacean taxa, including Volvox, are polyphyletic 35
2.4.4 There may have been several separate pathways leading from Chlamydomonas to VolvoX 37
2.5 The genetic program for cellular differentiation in Volvox should be amenable to analysis 38
3. ecological factors fostering the evolution of Volvox 40
3.1 Volvocaceans compete for resources in transitory, eutrophic waters 41
3.2 Even in permanent bodies of water, the volvocaceans flourish only briefly each year 42
3.3 Different aspects of the environment favor green algae of different body sizes 47
3.3.1 Smaller organisms have an intrinsic advantage under "optimum" conditions 47
3.3.2 Predation favors organisms above a threshold size 48
3.3.3 The larger the colony, the more efficient the uptake and storage of essential nutrients 50
3.3.4 In field studies, volvocacean ability to exploit eutrophic conditions has been found to be correlated with size 54
3.4 In eutrophic environments, differentiation of soma and germ is reproductively advantageous 55
3.4.1 Hypothesis: the soma acts as a source for nutrients, and germ cells act as a sink 56
3.4.2 Test: gonidia produce larger progeny in the presence of an intact soma and rich medium 56
3.5 Two major forms of source-sink relationship between soma and germ have evolved in Volvox 58
4. cytological features fostering the evolution of Volvox 62
4.1 The need to find a place in the sun imposes a rigid constraint on the structure of green flagellate cells 64
4.1.1 The photoreceptor uses calcium to signal light direction and intensity 65
4.1.2 The two flagella of Chlamydomonas respond oppositely to changes in calcium concentration 68
4.1.3 The difference between the two flagella is due to a difference in basal-body age 70
4.1.4 Proper orientation of the eyespot and the BBs must be maintained in each generation 72
4.2 Three kinds of cytoskeletal elements in the basal apparatus link BBs to other organelles 74
4.2.1 Microtubular rootlets link the BBs to the cortical cytoskeleton and the eyespot 75
4.2.2 Centrin-rich fibers connect the BBs to one another and to the nucleus 77
4.2.3 Assemblin-rich fibers connect the two halves of the BA to one another 80
4.3 Cell division in Chlamydomonas and related green algae has some unusual features 81
4.4 The BA plays a key role in segregation of organelles during division of C. reinhardtii 83
4.4.1 BBs are essential for coordination of karyokinesis with cytokinesis 84
4.4.2 The NBBCs couple the movements of the BBs to that of the mitotic spindle 85
4.4.3 MTRs maintain the spatial relationship between old and new BBs and define the cleavage plane 86
4.4.4 The role of the BA in cytokinesis assures orderly segregation of organelles 88
4.4.5 A new eyespot forms next to the new four-membered rootlet emanating from the younger BB 89
4.5 The BA and cell wall established the conditions that led to germ-soma differentiation 91
4.5.1 A coherent cell wall created a dilemma for green flagellates: "the flagellation constraint" 92
4.5.2 Small algae with coherent walls exhibit three solutions to the flagellation constraint 92
4.5.3 The presence of a Chlamydomonas-type wall is accompanied by multiple fission 94
4.5.4 Multiple fission facilitates colony formation 95
4.5.5 Larger algae circumvent the flagellation constraint in a new way: with sterile somatic cells 96
4.6 The extent of germ-soma differentiation increases with increasing size 97
4.7 Environment provided the stimulus and cytology provided the mechanism for germ-soma. 100
5. Volvox carteri: a Rosetta stone for deciphering the origins of cytodifferentiation 102
5.1 Some features recommending V. carteri f. nagariensis as a developmental model 103
5.2 Two formas of V. carteri differ greatly in their genetic accessibility 105
5.3 An overview of V. carteri anatomy and reproduction 109
5.3.1 Somatic cells are polarized, but gonidia are radial in organization 110
5.3.2 The BA is arranged very differently in somatic cells and gonidia 111
5.3.3 An asymmetric BA in somatic cells is essential for motility 112
5.3.4 A complex ECM holds the organism together 113
5.3.5 The asexual reproductive cycle is simple and rapid 114
5.3.6 The sexual cycle begins with a modified asexual cycle 115
5.4 Asexual morphogenesis and cytodifferentiation 117
5.4.1 Cleavage: creating two cell lineages from one 117
5.4.2 Inversion: turning the best side out 134
5.4.3 Cytodifferentiation: converting the germ-soma dichotomy from potential to actual 141
5.4.4 ECM deposition: architectural dexterity by remote control 148
5.4.5 Hatching: breaking up the old family homestead 161
5.4.6 Phototaxis: 4,000 oars responding to the commands of a single coxswain 164
5.4.7 Programmed somatic-cell suicide: the last full measure of devotion 172
5.5 Sexual induction and differentiation 174
5.5.1 The sources(s) of the sex-inducing pheromone 175
5.5.2 The nature of the sex-inducing pheromone 180
5.5.3 Developmental consequences of exposure to the pheromone 184
5.5.4 Biochemical effects of the pheromone and the riddle of its mechanism of action 188
5.5.5 Zygote formation and germination 202
6. Mutational analysis of the V. carteri developmental program 209
6.1 Formal genetic analysis 210
6.2 Drug-resistant mutants 213
6.3 Morphogenetic mutants 217
6.3.1 Cleavage mutants 217
6.3.2 Inversion mutants 225
6.3.3 ECM mutants 227
6.3.4 Cell-orientation mutants 230
6.4 Mutations affecting sexual reproduction 232
6.4.1 Constitutive sexuality 232
6.4.2 Reduced fertility and sterility 235
6.4.3 Male potency 237
6.4.4 Female hypersensitivity 238
6.4.5 Gender reversal 238
6.5 Mutations disrupting the normal germ-soma dichotomy 241
6.5.1 Somatic regenerator mutants 242
6.5.2 Late-gonidia mutants 246
6.5.3 Gonidialess mutants 247
6.5.4 Modifiers and suppressors 248
6.6 The genetic program for germ-soma differentiation: a working hypothesis 250
7. Molecular analysis of V. carteri genes and development 252
7.1 Some general features of the V. carteri genome and its replication 253
7.1.1 A curious uncertainty: Is nuclear DNA replication tied to the mitotic cycle? 256
7.1.2 Is all chloroplast DNA of somatic cells of gonidial origin? 260
7.2 Progress in establishing a DNA-based map of the V. carteri nuclear genome 261
7.2.1 Strains used for mapping DNA polymorphisms 261
7.2.2 DNA modifications complicate analysis of some V. carteri polymorphisms 263
7.2.3 RAPDs provide many additional markers 268
7.3 General features of V. carteri protein-coding genes 270
7.3.1 Exon-intron structure and the question of intron origins 270
7.3.2 The role of introns in regulating gene expression 274
7.3.3 Codon bias and its evolutionary implications 275
7.3.4 Putative regulatory signals 278
7.3.5 Volvox genes: plant-like or animal-like? 284
7.3.6 Gene duplication and/or homogenization 286
7.4 Development of molecular-genetic tools 287
7.4.1 Transformation 288
7.4.2 Reporter genes, inducible promoters, and anti-sense constructs 290
7.4.3 Introduction and expression of foreign transgenes 291
7.4.4 Gene modification by homologous recombination 293
7.4.5 Transposon trapping 294
7.5 Transposon tagging of developmentally important genes 296
7.5.1 Tagging and cloning of the regA locus 297
7.5.2 Tagging and cloning of a gls locus 299
7.6 Patterns of differential gene expression accompanying germ-soma differentiation 302
7.6.1 Early and late gene-expression patterns in somatic cells 302
7.6.2 Two patterns of gene expression in gonidia 304
7.6.3 Additional embryo-specific genes are readily identified 306
7.7 Patterns of gene expression in a mutant lacking germ-soma differentiation 307
7.8 Control of the germ-soma dichotomy: a new molecular-genetic hypothesis 308
Epilogue 313
References 319
Index
Preface
In the late nineteenth century, "development" and "evolution" were considered to be so intimately related that many biologists used the terms interchangeably, applying them rather indiscriminately to both the process that generates a new individual resembling its parents and the process that generates a new species different from its ancestors. For most twentieth-century biologists, continuation of that practice would have been unthinkable, because evolution and development have seemed to us to be such fundamentally different phenomena. However, history has a way of repeating itself. After a century-long estrangement that began with widespread rejection of Haeckel's dogma that "ontogeny recapitulates phylogeny," evolution and development are now undergoing a dramatic rapprochement, with genetics acting as the broker for their remarriage. Increasingly, those investigating the mechanisms by which differentiated cells and organs arise in the course of embryonic development and those seeking to understand how morphological novelties arise in the course of evolution find themselves converging on the study of related sets of genes, and talking to one another again!
Contemporary studies of Volvox, the rolling green spheroid that first fascinated Antoni van Leeuwenhoek 300 years ago, illustrate this sort of convergence. When my wife Marilyn and I began to study Volvox more than 20 years ago, our objective was to capitalize on its simplicity to address a central problem of development that we had found it extremely difficult to address with any clarity by studying vertebrate embryos: How do cells with very different phenotypes arise from the progeny of a single cell? But the longer we have worked with Volvox, and implored it to reveal its secrets to us, the more difficult we have found it to think about its development in other than an evolutionary framework. This is because it has become increasingly evident that the genes that Volvox now employs to establish a germ-soma division of labor during development exert their effects by modifying particular aspects of a genetic program and a set of cytological specializations that were inherited from ancestors (resembling modern Chlamydomonas and various colonial flagellates) that had only a single cell type. Therefore, the central premise of this book is that Volvox and its relatives provide us a unique window through which to examine the important roles that a few regulatory genes can play in both development and evolution, and gain fresh insights into a problem that has fascinated both developmental and evolutionary biologists for many generations: How do multicellular organisms with differentiated cell types arise from unicellular antecedents?
An effort has been made to write this book at a level that will be accessible to prospective biologists at the advanced undergraduate level and yet will be capable of holding the interest of more mature practicing biologists with a diversity of research interests. I would be less than forthcoming, however, if I did not admit to being motivated by an evangelistic spirit as I wrote, hoping to capture for an organism that I have come to love the vocational interests of young (or perhaps not so young) biologists who may be looking for a new window through which to view the living world.
In a very real sense, this book had its roots in a piece of advice given to me by my first mentor and scientific father figure, Jerome Gross (a piece of advice that other biologists caught up in the frenetic competitiveness of our current age might do well to consider). On the day that I was saying my farewells, about to leave for graduate school after spending a major portion of my undergraduate years in his laboratory, Jerry said, "If you want a happy and productive career in science, find yourself a quiet backwater where there are lots of big fish to be caught, but not many people fishing, and go after them." It took many years to find such a niche and begin to follow Jerry's sage advice.
It was Richard Starr who eventually led us to this niche, by introducing Marilyn and me to Volvox. For that, and for the friendship, help and encouragement that he has offered over all the intervening years - from the days he first hosted us in his laboratory and shared his cultures with us, up to and including his recent willingness to scrutinize a draft of this manuscript, point out various factual errors, and make many useful suggestions for improvement - I wish to express profound and lasting gratitude.
I also wish to thank two "life-history biologists" who introduced me to a completely new and different way of looking at Volvox and its relatives several years ago: Graham Bell and his then-student, Vassiliki Koufopanou. I was initially astonished at how we could look at the same set of simple organisms, see such different things, use such different lexicons to discuss them, and ask such different questions about their evolutionary origins. While I was consumed with a desire to understand the proximate (i.e., genetic and cytological) causes of the Volvox germ-soma dichotomy, they were consumed with a desire to understand its ultimate (i.e., ecological and adaptive) causes. Over the years the gap has been narrowed (and the minds broadened, I trust) by centripetal movement on both sides. Chapters 3 and 4 of this book constitute my attempt to incorporate into this narrative many ideas gained from interactions with Graham and Vasso, from their papers, and from the additional literature to which they directed me. Some of the original shortcomings of these chapters have been mitigated by suggestions they (particularly Vasso) made after reading a draft version. Thus, to the extent that I have succeeded in my effort to integrate into this book a consideration of the ultimate causes underlying the Volvox germ-soma dichotomy, credit goes to Vasso and Graham. But to the extent that I have failed, the fault is mine alone.
At the other end of the spectrum, the molecular end, an enormous debt of gratitude is owed to our good friend and close collaborator Rüdiger Schmitt and his colleagues. When Rudy and I met at a conference about 10 years ago and realized the extent to which we were attacking related aspects of Volvox biology from different perspectives, we drew up detailed plans for collaborative studies that have dominated the research agendas of both laboratories ever since. Little did we appreciate at the outset what a long-term program that would turn out to be, or how many obstacles would have to be overcome along the way. But as a result of this collaboration, we now have molecular-genetic methods for the study of Volvox that clearly would not yet exist if our two research groups had not worked together so closely over the past decade. Each major step along the way has been the product of a synergistic research relationship and has been enlivened and made much more enjoyable by frequent exchange visits between St. Louis and Regensburg by students, postdoctoral fellows, and technical assistants, as well as by Rudy and myself.
Above all, however, my gratitude extends to my wife and closest collaborator, Marilyn, without whom it is doubtful that Volvox research would still be alive and well in St. Louis, or in the larger community for that matter. For more than two decades she played the critical role of keeping our laboratory running efficiently during all the dreary hours when I was tied up with interminable committee meetings and other academic responsibilities. More importantly, she overcame the innate distaste of a classic biochemist for molecular biology - where one seldom can see a product, let alone weigh it - and was responsible for most of the critical breakthroughs required to bring Volvox research into the modern era. From the weekend on which (while cruising the northern Mississippi on an ancient stern-wheeler) we decided to visit Richard Starr and "go Volvox," to the present day (when she ignores her recent retirement and finds her way back to the bench whenever there is an important experiment that could benefit from her attention) it has been a productive relationship. I only hope that it has been half as rewarding and enjoyable for her as it has been for me. Her painstaking and critical reading of every word of this text, in each of several versions, has engendered both my sympathy and gratitude and has deepened my affection.
Finally, I wish to thank the National Institutes of Health, which supported our Volvox research over many years, the National Science Foundation, which has done so in recent years, and NATO, which provided travel grants to Rudy Schmitt and myself to facilitate our transatlantic collaboration.
Prev Page | Home Page
SOCIETY FOR DEVELOPMENTAL BIOLOGY
SDB Webmaster
Posted Thursday, January 7, 1999